Back to the Future: Beyond the Gasoline Age
The Race to Replace the Gas Tank: Which Technology Will Win the Race to Power the Cars of Tomorrow? Why Your Next Car Might Not Be What You Expected?
TL;DR
No single technology emerges as a perfect solution – each has distinct advantages and limitations.
Environmental impact is still massive, even with EV. The car emission is only one aspect, as producing an EV is much more polluting than a standard car.
Geopolitical forces also take a significant part here, in many aspects. From critical mineral supply, battery supply chain, and just political influence.
You should take a look at sodium-ion and hydro fuel cell technologies. They both suffered from a rough start, but their potential is huge with the right infrastructure and further tech optimizations.
“Where we're going, we don't need roads” (Back to the Future, 1985)
Introduction
Cars have always been a cornerstone of modern life, offering freedom and mobility while leaving behind a trail of environmental challenges. They symbolize freedom, status, and progress, yet their impact on the planet is impossible to ignore. Another issue which is still minor but we all know is coming - the oil won’t be here forever, so we must be prepared toward that day. It’s estimated that known oil deposits will run out by 2052, and different financial institutions as Goldmans-Sachs and McKinsey predict the peak demand will be between 2029 to 2034, reaching 110m barrels per day, which will shift the price based on demand and supply.
While autonomous vehicles often grab headlines, a more critical revolution is happening under the hood – one that will shape how we power our journeys in the years ahead. For over a century, internal combustion engines (ICEs) have driven our vehicles, fueling economic growth but also contributing significantly to global emissions. In the past decade, electric vehicles (EVs) have surged in popularity, offering zero tailpipe emissions and rapid advancements in battery technology. Yet, EVs come with their own challenges: reliance on rare earth minerals, the environmental toll of battery production, and the need for widespread charging infrastructure.
The idea of a single, universal solution is a myth. While compact EVs may be perfect for urban commuters in cities like New-York, Oslo or Singapore, they might not meet the needs of long-haul truckers crossing the Australian outback or farmers in rural India. Different technologies—from hybrids and hydrogen fuel cells to biofuels and even improved ICEs—will likely play roles tailored to diverse needs.
The future of car engines will be as varied as the communities they serve. In the sections ahead, we’ll explore the strengths and limitations of these technologies, uncovering how they might coexist to drive us toward a more sustainable, greener, and basically a better future.
Types of Car Engines
Remember those toy cars you'd pull back and watch zoom across the floor? Real cars work on similar principles – they just need energy to make the wheels turn. Let's look at the different ways we can provide that energy, and what each means for our world.
Internal Combustion Engine (ICE)
How it works: Internal combustion engines burn fossil fuels like gasoline or diesel to create energy. The combustion process generates heat, which powers pistons that move the vehicle. In other words, it mixes fuel with air and creates thousands of tiny explosions to power your car.
Advantages:
Well-established technology with a global refueling infrastructure.
Capable of delivering high power and range.
Challenges:
High carbon dioxide (CO2) and pollutant emissions.
These engines still waste about 60% of their energy as heat. Despite improvements in efficiency, only 20%-40% of the fuel energy is actually converted into useful mechanical work.
Dependence on finite fossil fuels that will eventually run out.
Increasing regulations on emissions standards make ICEs less viable long-term.
Hybrid and Plug-in Hybrid (HEV & PHEV)
How they work: Hybrids combine a traditional ICE with an electric motor and battery. Plug-in hybrids have larger batteries that can be charged externally, enabling short trips on electric power alone. When you need lots of power (like climbing hills or accelerating), both systems work together. When you need less power, the electric motor can take over, saving fuel. The clever part? The car recaptures energy usually lost during braking to charge its battery. Plug-in hybrids take this further by letting you charge the battery from your home outlet, potentially covering your daily commute on electricity alone.
Advantages:
Improved fuel efficiency by switching between electric and gasoline power.
Reduced emissions compared to ICEs, especially in city driving.
Extended range, with the flexibility of gasoline for long trips. (no range anxiety)
Challenges:
Higher initial cost compared to ICE vehicles.
Dependence on fossil fuels for extended trips.
You're still carrying two systems, which means more weight and complexity for maintenance.
Battery Electric Vehicles (BEVs)
How they work: Electric cars are surprisingly simple – they're basically big RC cars. An electric motor (or several) powered by a large rechargeable battery pack drives the wheels directly. No explosions, no exhaust pipe, no oil changes. When you press the accelerator, power flows instantly to the wheels, no delay, no gear shifting, just instant acceleration.
Advantages:
The car has zero tailpipe emissions, contributing to cleaner air.
Increasingly cost-competitive as battery technology improves.
Challenges:
Limited range and long charging times compared to ICEs.
Batteries are the most heavy and expensive part in the car production.
Battery production depends on rare earth minerals like lithium, cobalt, and nickel, whose mining harms ecosystems and communities.
Requires a robust charging infrastructure, and efficient electricity grid (which usually is decades old).
Shift the initial energy production from the car owner to the authorities.
Hydrogen Fuel Cell Vehicles (FCEVs)
How they work: Imagine a power plant so clean its only waste product is water. That's what hydrogen fuel cells offer. FCEVs use hydrogen gas and oxygen to produce electricity through a chemical reaction in a fuel cell, which powers the vehicle’s electric motor.
Advantages:
High efficiency and zero tailpipe emissions (only water vapor is emitted, which is basically the best outcome by far).
Fast refueling times, similar to traditional ICE vehicles.
Longer range compared to BEVs.
Similar technology can be used in all transportations vehicles and in other industries as well.
Challenges:
Hydrogen is tricky to store and transport, as it’s flammable, and requires extra measure.
New infrastructure will need to be built (like building a network of hydro gas stations, even embedding them in existing ones).
Expensive and sparse hydrogen production. Production phase requires lots of energy, and will require optimizations.
Low adoption rates due to high vehicle and fuel costs.
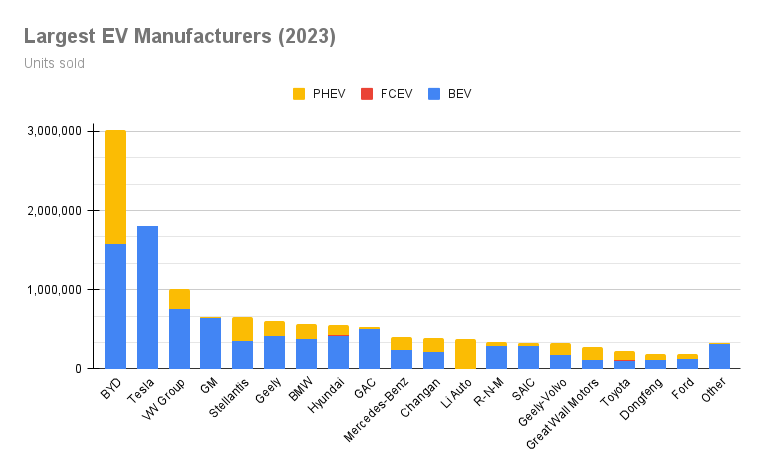
Other Niche Technologies
While electric and hydrogen vehicles dominate discussions about the future, several other technologies offer interesting alternatives. Though they may not become mainstream solutions, they showcase the diverse ways we can approach cleaner transportation.
Natural Gas Vehicles
Natural gas vehicles (NGVs) use compressed natural gas (CNG) or liquefied natural gas (LNG) as fuel, combusting it in a modified internal combustion engine. In other words, these cars run on the same gas that heats many homes, but in compressed form. They produce fewer emissions (CO2) than gasoline engines and the fuel costs less, depending on the region and time (remember the gas price when Russia invaded Ukraine?). However, limited fueling stations and the need for large fuel tanks have kept these vehicles mainly in fleet operations like buses and delivery vehicles. And also, it’s still a fossil fuel with methane, not exactly a solution for the long run.
Biofuels
Imagine powering your car with fuel made from corn, sugarcane, or even used cooking oil. That's the idea behind biofuels. While they can work in modified traditional engines and produce less net carbon emissions, they face criticism over using farmland for fuel instead of food. I will avoid entering this philosophical discussion, but studies show that around 15.3% of global food production is wasted annually, most of it in middle and high-income countries. Also, there is a gigantic percentage of the crops going to waste, and also around 36%-41% of the global crop products goes to animal feed and not directly to humans, so it’s a bit more complex issue with many aspects. As for today, most commonly, this technology is used as additives to regular gasoline rather than pure fuel.
Synthetic Fuels
These are artificial fuels created by combining hydrogen with captured carbon dioxide. The appeal? They work in regular engines without modification (potentially can reach net-zero emissions if produced using renewable energy). The downside is the high production cost and energy-intensive manufacturing process, making them currently practical only for specialized uses like motorsports.
Solar-Assisted Vehicles
While cars powered entirely by solar panels remain impractical (they need too much surface area), solar assistance is finding its niche. Small solar panels on vehicles can help power auxiliary systems or provide additional charge to hybrid and electric vehicles. Think of it as a small helper rather than a main power source – useful for extending range slightly or running climate control while parked. Might also be more practical in large vehicles such as trucks and trains for supporting demanding systems such as cooling.
The Winner Takes It All: The EV is leading the way. For now.
Believe it or not, the electric car was already invented in Scotland between 1832 and 1839 by Robert Anderson, but to be fair it was an electric carriage, powered by non-rechargeable primary cells. This early prototype could travel at around 12 km per hour and used crude oil to generate electricity. During the following decades it got more technological breakthroughs, including rechargeable batteries (we’re still in the 19 century, yes?), and although there were few commercial successes, history shows people chose gasoline for the following century, until recently.
Battery Electric Vehicles (BEVs) have emerged as the dominant alternative to Internal Combustion Engine (ICE) vehicles, driven by advancements in battery technology, growing environmental awareness, and supportive government policies.
Current State of BEV Technology
Market Growth and Penetration: Global BEV sales are expected to reach 15.1 million units in 2025, a 30% increase from 2024, accounting for approximately 16.7% of global light vehicle sales. China leads the market with 65% of global EV sales in 2024, followed by Europe and the U.S.
Major Players: Tesla, BYD, and legacy automakers like Volkswagen and General Motors are leading the BEV market. Tesla continues to dominate with its advanced battery tech and extensive charging network, while Chinese automakers like BYD are rapidly expanding globally, offering a wide variety of cars for a more affordable price. Volkswagen aims to become the world's largest EV maker by 2025 (good luck with that), while GM plans to go all-electric by 2035. There is no point comparing the companies’ market value since BYD deliver much more cars, but Elon Musk close ties with Trump created an increase of Tesla stock value, regardless to the company’s performance.
Sales Projections: By 2030, BEVs could account for up to 45% of global light vehicle sales, driven by declining battery costs and stricter emissions regulations.
Range Improvements and Charging Innovations
Range Advances: Early EVs faced criticism for limited driving ranges (causing a phenomenon called “range anxiety”), but the modern BEVs now offer ranges between 400-600 km per charge, with some models expected to surpass 1,000 km by 2026 due to innovations like solid-state batteries and silicon-anode technologies.
Charging Innovations: Slow charging is the achilles heel of the EV. The car owners know that the notorious Level1 charging (standard 110/120V) takes over 24 hours, and level2 (using a 7kW charger) which is the most popular on homes, work and public, it can average takes can take 6-8 hours (with Hyundai Ioniq Electric and MG5 EV), sometimes more (Tesla Model S) and sometimes less, depending on the type of battery and the electricity infrastructure.
Like in many smartphones, fast charging techniques (50kW) became more popular providing 80% in about 40 minutes for most EVs, and ultra-fast chargers (150kw) can achieve around 80% charge in 18-30 minutes. Other solutions for ultra-fast charging come from companies like CATL which deliver over 600 km of range in just 10 minutes. Additionally, smart charging technologies and Vehicle-to-Grid (V2G) systems are being integrated to optimize grid usage and reduce costs, making BEVs even more appealing and practical for everyday use.
Still slower than fueling, no real standards, but the positive side is you can charge the car while you’re away.
Lithium-Ion Batteries: The Core of BEV Success
Lithium-ion batteries are the cornerstone of current BEV technology. You may already know it as the technology of the battery in your phone or your laptop. The lithium-ion batteries offer a high energy density, long lifespan, the ability to recharge relatively quickly, and the battery costs have dropped significantly over the past decade, making them the optimal choice for powering modern EVs. However, this dominance comes at a cost. However, this technology comes with significant environmental trade-offs that often go unnoticed in discussions about "zero-emission" vehicles.
The Environmental Paradox
I haven't studied chemistry since high-school, so please forgive me if not all the terms will be accurate, but since we’re looking at the business side of this, you will get the idea of why we face a problem.
According to GreeCars Expert, a typical EV battery contains approximately:
17 pounds of lithium carbonate
77 pounds of nickel
44 pounds of manganese
30 pounds of cobalt
That's more or less the secret recipe, although there are always new developments in lithium batteries (like LFP batteries), usually with different ratios and sometimes changing some of the ingredients with other materials.
Now let’s focus on the rare (and expensive) minerals in this list: Lithium, Nickel and Cobalt.
For converting raw minerals into products we usually have 3 stages: mining, refining, and production (or manufacturing). Each phase has its own characteristics, requirements, and impact on the environment. Some of them will make you wonder how the hell we are still using these minerals.
From the business point of view, the demand for these minerals keeps growing only one way, the price goes up (dramatically), so more players get into the business of mining (sometimes with new technologies, sometimes just powerful people with special interest), production is high, price goes down, consolidation in the industry, and all over again. The EV maker needs to manage its supply chain well in order to lower the risks of volatility of raw materials, but eventually it affects the prices of the battery and the finalized EV car, mostly one way and it’s not down. (it’s capitalism after all)
So let’s dive into the details.
Lithium
Current Price is around around 75,500 CNY per ton (around $10,230 USD).
It reached an all time high of 575,000 CNY (approximately USD 80,000) in late 2022.
75% of lithium comes from the "Lithium Triangle" of South America - Chile, Bolivia, and Argentina. Other important players are China and Australia. More thoughts on that will be later on, on the geopolitics aspect.
Cobalt
Currently priced at $24,300 USD per ton.
Its peak price reached around $95,250 USD in early 2018.
Cobalt remains one of the most expensive battery metals, with 70% of global production concentrated in the Democratic Republic of Congo. The mining often involves hazardous working conditions and environmental degradation.
Nickel
Maintained relatively stable prices around $15,200 USD per ton.
Although it has a spike during late 2022 (like all Cobalt and Lithium), it shows less volatility compared to the other minerals.
Indonesia, Australia, and Brazil dominate nickel reserves, although its mining and refining capabilities do not yet reflect the full potential.
A quick conversion of the battery recipe above, and now we can see the big money is on Cobalt and Nickel (while lithium had its moments).
17 pounds of lithium carbonate = 17/2000 * 9,100 = $774
77 pounds of nickel = 17/2000 * 15,400 = $5,929
44 pounds of manganese = 44/2000 * 1,494 = $329
30 pounds of cobalt = 30/2000 * 19,989 = $2,998
This reflects only the current value of raw materials, there are so many other moving parts that accumulated into the final EV car value.
Sodium-ion: is it the game changer we waited for?
As the limitations of lithium-ion batteries become more apparent, sodium-ion technology is gaining attention as a potential alternative, especially for EVs. Sodium-ion batteries promise significant benefits over their lithium-ion counterparts but come with their own challenges.
Benefits of Sodium-Ion Batteries:
Faster charging capabilities, potentially reaching 80% capacity in under 15 minutes with the current technology.
Superior performance in cold weather, maintaining better capacity than lithium-ion batteries below freezing.
Greater stability and safety, with lower risk of thermal runaway (battery fires).
More environmentally friendly production and disposal processes
Sodium is far more abundant and cheaper to source than lithium, reducing production costs, as well as reducing supply chain risks.
Sodium-ion batteries use materials that are less hazardous to extract and handle, making them more environmentally friendly.
Current Status and Limitations:
Sodium-ion batteries currently have a lower energy density compared to lithium-ion, meaning they store less energy per kilogram. This means either accepting shorter ranges or using larger, heavier battery packs. However, researchers predict sodium-ion technology could reach 200 Wh/kg by 2025.
The technology is still in its infancy, with limited commercial availability and ongoing research to improve performance.
Examples in Use: Several Chinese automakers have begun incorporating sodium-ion batteries into their vehicles. For example, JAC Motors and Chery have announced plans to use sodium-ion batteries in their compact EV models, targeting cost-conscious consumers and regions where affordability is a key concern. BYD, the world's largest EV manufacturer, announced plans to mass-produce sodium-ion batteries in 2024 in Xuzhou. Stellantis invested in French battery maker Tiamat for sodium-ion technology development.
As sodium-ion technology matures, it may not entirely replace lithium-ion batteries but could complement them, especially in affordable and regional EV markets. We might see hybrid batteries solutions, we might see different car types for different areas and use-cases. It's particularly well-suited for:
Affordable city cars where range requirements are modest.
Cold-climate regions where performance advantages matter more than maximum range.
Energy storage systems where cost matters more than size and weight.
While EV battery technologies are advancing, they are not without drawbacks. Both lithium-ion and sodium-ion solutions rely on rare minerals, and their widespread adoption shifts a significant part of the energy production burden from individual car owners to governments and power grids. This is especially concerning in an era where electricity demands are surging due to crypto mining and AI technologies, both of which require immense computational power. These trends intensify the challenges of electricity production, which is neither unlimited nor always environmentally friendly—a topic that warrants separate analysis.
Best of Both Worlds: The Hybrid Revolution
While electric vehicles grab headlines and traditional gas engines maintain their familiar presence, hybrid vehicles have quietly proven themselves as the practical middle ground. Their success lies not in revolutionary technology, but in clever engineering that maximizes the strengths of both electric and gasoline power.
Modern hybrids achieve their impressive efficiency through sophisticated power management. Take the latest Toyota Camry Hybrid: it delivers 52 miles per gallon in city driving – double the efficiency of its gas-only sibling. This isn't just about fuel savings; it's about using the right power source at the right time. In stop-and-go traffic, the electric motor handles most of the work. At highway speeds, the gas engine takes over at its most efficient range. When you need maximum power, both systems work together.
Real-world efficiency tells the story:
A conventional Camry uses 4.8 gallons of gas to cover 100 miles of city driving.
The hybrid version uses just 1.9 gallons for the same journey.
Hybrid (HEV) vs. Plug-in Hybrid (PHEV): A Key Distinction
While conventional hybrids rely on regenerative braking and the ICE to recharge their batteries (I admit it’s quite an elegant solution), there are the rising plug-in hybrids (PHEVs) that take this a step further by allowing external charging. This gives PHEVs a larger battery and an extended electric-only range—often 20-50 miles—making them ideal for short daily commutes without using gasoline. However, for longer trips, the ICE seamlessly takes over, eliminating any potential for range anxiety.
For this car you will need a charging infrastructure, as any BEV, but you won’t be dependent on it.
The downside is that Plug-in Hybrid is usually the most expensive option out there, more than hybrid (HEV) and more than many of the BEV out there. The premium price is affected by their dual powertrain technology, and by growing demand that faces a cautious production strategy.
And yes, it does have 2 holes ports, one for charging (level 1 and level 2), and a standard fuel filler port.
Market Trends
The HEV has 9.6% of the total light-duty market, while the PHEV is around 2.0%. Although it’s still small, the PHEVs are experiencing a significant growth, particularly in China with 98% year-over-year increase, and is expected to grow globally by 75% in 2024 compared to previous year, reaching over 7m units.
The largest market with 20.4 of PHEV units by 2023 is China, while Europe is the second-largest market with 11.8 vehicles. The US has sold only 4.74 PHEV since 2010.
It’s interesting to remember that hybrid cars were available in the market much before EV became a commodity, and no one knew about Tesla and electric cars were just geek’s fantasy. Unlike what I expected to find, the EV is maybe a trend in the news, but the numbers are telling a different story, more complex. Different countries, encouraged by subsidies and efficient marketing (and maybe environmental awareness), have different ratios between cars segments. When asking for consumer preferences, the US is still full power on gasoline, but also more hybrid than expected. China, as expected, keeps its faith to their EV industry, while Japan, South-Korea and India have the significant segment goes for Hybrid.
Success Stories: Toyota's Ongoing Hybrid Leadership
No discussion of hybrids is complete without acknowledging Toyota's role. Since launching the original Prius in 1997, Toyota has sold over 20 million hybrid vehicles globally. Their persistence with hybrid technology, even as others focused solely on pure electric vehicles, has paid off:
The Prius became the world's best-selling hybrid, creating a new vehicle category
Toyota's hybrid technology now spans their lineup, from compact cars to luxury SUVs
Their hybrid vehicles have prevented over 160 million tons of CO2 emissions compared to equivalent gas-powered vehicles
This success stems from focusing on practical benefits rather than revolutionary promises. While hybrids may not be the ultimate solution for a sustainable future, they play a crucial role in reducing emissions and easing the transition to full electrification. By blending the strengths of ICE and EV technologies, as well as battery technology improves and charging infrastructure expands, hybrids embody a pragmatic approach to meeting diverse consumer needs and environmental goals.
Hydrogen: A Dark Horse or the Future King?
Hydrogen fuel cell technology offers an elegant solution to many of the challenges faced by traditional internal combustion engines (ICE) and battery electric vehicles (BEVs). With water as its only exhaust product and fast refueling capabilities, hydrogen is being championed as a potential game-changer in the race for cleaner transportation.
The Elegance of Hydrogen Power
Hydrogen fuel cells (FCEV) convert hydrogen gas into electricity through a chemical reaction, emitting only water vapor as a byproduct. This technology offers efficiency comparable to or better than ICE vehicles while maintaining the convenience of quick refueling—often under five minutes—making it an attractive option for consumers accustomed to gasoline cars.
Hydrogen’s high energy density makes it particularly well-suited for long-range and heavy-duty applications, where battery limitations become more apparent. This includes: long-haul trucks, buses and fleet vehicles, maritime vehicles.
Is Hydrogen Dangerous?
Despite common misconceptions, hydrogen-powered vehicles are not akin to hydrogen bombs. While hydrogen is highly flammable, modern fuel cell vehicles are equipped with robust safety measures, including reinforced tanks and advanced leak detection systems. These safeguards ensure that hydrogen cars are as safe as, if not safer than, traditional gasoline vehicles.
The Hydro Challenges
While hydrogen fuel cell vehicles (FCEVs) offer promising environmental benefits, the technology faces substantial challenges that hinder widespread adoption. The production of fuel cells remains expensive, largely due to their reliance on precious metals like platinum and iridium as catalysts, accounting for up to 24% of stack costs.
Infrastructure development presents another critical barrier, with fewer than 80 hydrogen refueling stations in the United States, mostly concentrated in California, compared to over 105,000 EV charging stations.
Storage challenges persist due to hydrogen's unique properties - it requires complex high-pressure tanks (700 bar) or cryogenic systems, making vehicle design and safety considerations more demanding.
Public awareness and acceptance also remain obstacles, with studies showing that less than half of the population in major markets are familiar with hydrogen vehicle technology (I was assuming the real number is under 20% or even 10%).
The technology suffers from a "chicken-and-egg" dilemma: without sufficient refueling stations, consumers hesitate to buy FCEVs, but without enough vehicles on the road, investing in stations is difficult to justify. Additionally, the overall cost of hydrogen fuel production remains high, ranging from $3.00 to $6.55 per kilogram using renewable resources, making it less competitive compared to other alternative fuel options, and as we all know, it’s always the economics.
Business Aspects and Market Leaders
The global hydrogen vehicle market presents a complex business landscape dominated by Asian manufacturers. Japan and South Korea lead the charge, with Toyota and Hyundai at the forefront of FCEV development. Japan aims to boost its annual hydrogen supply to 3 million tonnes by 2030 and 20 million by 2050, backed by substantial government investment of 15 trillion Japanese Yen (over $100 billion). South Korea demonstrates similar commitment, with five major conglomerates pledging approximately $33 billion through 2030 for hydrogen infrastructure development.
However, recent market performance reveals significant challenges. Toyota's Mirai and Hyundai's Nexo saw disappointing sales in 2023, with only 2,737 and 241 units sold respectively in the US. Japan experienced an 83% decline in hydrogen car sales over two years, with merely 422 units sold in 2023. Despite these setbacks, major automakers continue their investment in hydrogen technology. BMW recently announced plans to launch its first production hydrogen vehicle by 2028, partnering with Toyota for fuel cell technology.
The U.S. government has attempted to stimulate the market through the Inflation Reduction Act's tax credits and $8 billion investment in hydrogen hubs. However, infrastructure remains a critical bottleneck, with California's hydrogen highway facing significant challenges as station owners abandon operations. Claims about Tesla developing a "Model H" hydrogen vehicle by 2026 have been definitively debunked by fact-checkers, with Elon Musk maintaining his skeptical stance toward hydrogen technology for passenger vehicles (just don’t forget he is heavily invested in batteries, and very unexpected in general).
So to conclude this part, hydrogen’s adoption faces a classic chicken-and-egg dilemma: infrastructure development lags due to low vehicle adoption, while limited adoption persists because infrastructure is sparse. Despite these challenges, its unique advantages make it a promising contender in the future of transportation. By addressing current challenges, keeping the innovation, but mainly getting governmental decisions done as tax cuts or subsidies to encourage expanding of the infrastructure, hydrogen could finally play a critical role in reshaping the automotive landscape.
The Geopolitical Game
The transition to alternative vehicle technologies has created a complex web of geopolitical interests, power struggles, and environmental challenges that are reshaping global relationships and economic structures.
Why China?
China's aggressive push into electric vehicles goes beyond environmental concerns. Despite being the world's largest CO2 emitter, China has strategically positioned itself as the global leader in EV production, accounting for 65% of global sales in 2024. This dominance serves multiple objectives: reducing urban pollution, decreasing oil dependency, and establishing technological supremacy in the automotive sector. China's manufacturing prowess and control of critical battery materials has created a cost advantage that Western markets are struggling to counter. During the past years it strengthened its economic ties with different countries in Africa in order to secure its resources, and the execution was well done.
Chinese leaders know that switching all the cars won’t reduce enough CO2 emissions, as there are lots of power plans and heavy industry that contribute too much, but maybe finally the cities skyline will be clearer, and it will also reduce the risk of a crisis in their health system due to related health problem (and during Covid-19 they got a reminder how fragile their health system could be).
The Hydrogen Race in East Asia
Japan and South Korea have taken different paths, focusing heavily on hydrogen technology. South Korea aims to produce 300,000 FCEVs and 21,200 buses by 2030, while Japan leads in hydrogen infrastructure development and has pioneered commercial hydrogen vehicles through Toyota. Both countries are pursuing international partnerships, as evidenced by their first Korea-Japan Hydrogen Cooperation Dialogue in June 2024, demonstrating their commitment to establishing hydrogen as a viable alternative to battery electric vehicles. Western countries for now stay more suspicious with this technology, and maybe it’s also because of the variety of alternatives.
The Mining Economy Paradox
The mining sector creates a complex economic dependency in developing nations, where mineral wealth often breeds both opportunity and vulnerability. This dependency has shaped the economic landscape of several key nations in distinctly different ways.
The Democratic Republic of Congo (DRC) exemplifies extreme mining dependency, with the sector contributing over 25% to GDP and representing 95% of exports. While mining generates around $1 billion in revenues and sustains approximately 10 million people through artisanal mining, the country struggles with systemic corruption and illegal mining. An estimated 98% of artisanal gold production is smuggled out of the country, representing massive losses in potential tax revenue.
Chile's mining sector, more structured but equally vital, represents 13.6% of GDP and 58% of total exports. The industry controls 24% of global copper production and creates significant economic ripples - every $100 in mining generates an additional $36 in indirect economic activity. Argentina, another output of economic failure and corruption, has an emerging mining sector, presents a smaller but growing presence, contributing 0.82% to GDP while providing wages 1.8 times higher than the national average.
A sudden cessation of mining operations would trigger catastrophic consequences. Immediately, it would eliminate approximately 4 million formal mining jobs globally and impact over 100 million informal livelihoods. Government revenues would face severe shortfalls, particularly in the DRC where mining provides 40% of state income. Long-term consequences would include economic crises in mining-dependent regions, social instability from unemployment, and infrastructure development slowdown.
These nations are caught in a "mining trap" - dependent on mineral extraction for economic survival while struggling to translate this wealth into sustainable development and economic diversification. The challenge lies not just in managing these resources effectively, but in using them as a stepping stone toward more resilient economies. If you think it was not done correctly, you are probably right.
The Legacy Mine Cleanup Crisis
The challenge of cleaning abandoned mines presents a growing environmental and financial burden. In the United States alone, cleaning up abandoned hardrock mines has cost taxpayers $119 million over just four years (2017-2021). The total cleanup cost remains unknown but is estimated to be in the billions. This raises serious questions about responsibility and funding, as many mining companies declare bankruptcy or abandon sites without proper remediation. Now let’s imagine what will happen in countries like Chile, The Democratic Republic of Congo (DRC), and you get the point.
Oil's Persistent Influence
Traditional oil powers are navigating the transition while trying to maintain their geopolitical influence. OPEC+ countries continue to use production decisions as a political tool, while the United States has achieved record oil production under both Trump and Biden administrations despite their different energy policies. The geopolitical importance of oil remains evident in Middle East tensions, where conflicts could potentially drive prices above $100 per barrel, which makes more western countries (and China) to have a special communication channel with countries like Saudi-Arabia, Kuwait and Qatar, which give them a great influence as well as “causing” a short memory of crimes, double dealing and human rights issues.
This complex landscape illustrates how the transition to alternative vehicle technologies is not just a technological challenge but a fundamental reshaping of global power dynamics, economic relationships, and environmental responsibilities. Countries that have a long run strategy should jump on this opportunity in order to become the next superpower. Either way, it will be interesting.
The Bigger Picture: Environmental Aspects of Clean Cars
The promise of "clean cars" often conjures images of a greener, more sustainable future, but the reality is far more complex. While alternative vehicle technologies like EVs and hydrogen fuel cells aim to reduce emissions, they also introduce new environmental challenges that must be critically examined. Here’s a closer look at the broader environmental picture.
“Clean Cars” vs. “Clean Energy”
While electric vehicles produce zero direct emissions, they effectively transfer the environmental burden to power plants. However, this shift actually represents a net benefit - even when powered by coal-heavy grids, EVs produce fewer total emissions than gasoline vehicles due to the higher efficiency of large-scale power generation. The average EV in the US produces emissions equivalent to a hypothetical 94-mpg (miles per gallon) gasoline car, demonstrating that the centralization of emissions is not a sustainability scam but rather an efficiency improvement. It also depends on the energy source mix of the grid, how much of it is powered by coal, natural gas, oil or renewable energy.
Raw Material Sustainability
The production of EV batteries raises serious sustainability concerns.
We mentioned earlier in this article the need for critical materials like lithium, cobalt, nickel, and graphite face supply constraints and environmental challenges in extraction. From the initial extraction process until the final output we need to go over 3 steps: mining, refining, manufacturing (or producing).
Let’s dive into some aspects of each step:
Mining Impact:
Extracting lithium from brines requires vast amounts of water—up to 500,000 liters per ton—leading to water scarcity in arid regions like Chile's Atacama Desert (lithium mining uses 65% of the region’s water resources).
Mining processes release toxic chemicals into soil and water systems, harming ecosystems and local communities.
Nickel mining causes deforestation of 378,970 acres in Indonesia alone.
Mining one ton of lithium generates approximately 15 tons of CO2 emissions due to energy-intensive extraction methods. Not exactly eco-friendly.
Deep-Sea Mining: Last Week Tonight with John Oliver (HBO)
Episode aired Jun 9, 2024.
Refinery Impact:
Lithium refinery requires extremely high temperatures (800-1000°C), and most of it takes place in China that rely on coal-powered electricity. Ironically this process consumes a large amount of energy.
The refinery of most minerals produces massive amounts of toxic waste, which also poses risks of workplace exposure to toxic substances.
Often results in submarine tailings disposal.
Manufacturing Impact:
According to McKinsey, Producing an electric vehicle currently generates more emissions than manufacturing a conventional car, primarily due to battery production (around 40%-60% during a single EV production).
A typical 75 kWh battery pack might generate 4-5 tons of CO2 during manufacturing – equivalent to driving a gasoline car for several months.
Recycling Challenges:
While lithium-ion batteries are recyclable, only about 5% of lithium-ion batteries are currently recycled due to high costs and technological barriers, as the process is complex and energy-intensive. Current recycling methods recover only 50-60% of materials, though new technologies promise higher recovery rates.
The industry faces a growing urgency to develop more efficient recycling solutions as the first generation of mass-market EVs approaches end-of-life.
Grid Infrastructure Challenges
The shift to EVs (and plug-in hybrids) places enormous pressure on aging grid infrastructure. Most power grids were not designed to handle the sudden surge in electricity demand from millions of EVs charging at the same time. Without significant upgrades, grids may face severe stress, particularly during peak charging periods, leading to scenarios as blackouts or brownouts.
Additionally, current grids suffer from inefficiencies, with energy losses occurring during transmission from power plants to end users. This reduces the net efficiency of EVs compared to traditional ICE vehicles, which rely on localized energy production (in other words - we need to produce more electricity than we are actually going to use). Renewables like solar and wind, while promising, pose integration challenges due to their intermittent nature, necessitating large-scale storage solutions. These solutions, however, demand more raw materials like lithium, adding to existing supply chain pressures. (let’s hope we could use the sodium-ion based storage solution if we prefer to go greener, but I don’t trust this will be the case)
Net Environmental Impact
Despite these challenges, and personally it was despite my suspicion, studies show that lifecycle assessments reveal encouraging results.
EVs reduce lifetime greenhouse gas emissions by 52% compared to gasoline sedans. The environmental break-even point occurs within 1-2 years of typical driving in most regions. Manufacturing emissions are higher for EVs initially but are offset by operational benefits.
There are many factors to take into account, and every new piece of information (and especially predictions!) you should take it with a grain of salt. For example, did the previous research take into account only CO2 emission or all the extra damage which is caused during mining and refining? It’s impossible to track it in such a high resolution for long, maybe the whole calculation is impossible. But in high level, what will eventually make it net positive or net negative are the following:
Global Energy Transition: The speed at which grids can transition to renewable energy sources.
Technological Advancements: Innovations in battery recycling, raw material alternatives, and energy efficiency.
Policy and Incentives: Government regulations and subsidies that promote sustainable practices across the supply chain.
Ultimately, the path to sustainable transportation demands a holistic approach—one that addresses not only vehicle emissions but also the environmental and societal costs embedded in their production and operation. While "clean cars" may not mean zero environmental impact, they are a vital piece of the puzzle, and they cannot be the whole solution without a concurrent transformation of our energy and industrial systems.
ReAssemble
The future of automotive propulsion isn't a race to crown a single winner, but rather an evolution toward a diverse ecosystem of technologies. Each solution—whether electric, hybrid, or hydrogen—brings unique strengths and limitations to address different transportation needs
The Myth of a Perfect Solution
No single technology offers a complete answer to our transportation challenges. While EVs dominate headlines with their zero tailpipe emissions, they shift environmental impacts to battery production and power generation. Hydrogen promises clean operation but faces infrastructure hurdles. Hybrids offer practical compromises but still rely partially on fossil fuels.
Regional Realities
Transportation needs vary dramatically across regions and use cases. Dense urban centers may thrive with battery electric vehicles, while rural areas might benefit more from hybrid or hydrogen solutions. Commercial transport, particularly long-haul trucking and heavy machinery, requires different solutions than personal mobility
The Innovation Imperative
Competition between technologies drives innovation across all platforms. Battery improvements benefit both pure EVs and hybrids. Advances in hydrogen fuel cells could revolutionize heavy transport. This technological diversity creates resilience and speeds development across the entire sector.
Policy and Infrastructure
Looking ahead, policy and infrastructure will play a critical role. Governments must prioritize renewable energy grids, efficient charging networks, and incentives for sustainable practices. Industries should innovate responsibly, addressing the life cycle of vehicles from production to recycling.
Emerging technologies like sodium-ion batteries and advances in hydrogen production present exciting opportunities, but their success depends on overcoming economic and logistical hurdles. Achieving sustainable transportation will require collective effort across industries, governments and consumers, all recognize that clean cars are just one piece of the larger puzzle of sustainable living.
The journey forward is complex but hopeful, grounded in the idea that by embracing diversity in solutions, we can accelerate toward a cleaner, more sustainable future.
And for me, I like to hear my engine roars 🫣
The race to decarbonize electric-vehicle batteries, McKinsey & Company, February 23, 2023.
Energy Transition in Lithium Ion Batteries and the Impact on the DRC, GeopoliticsUnplugged, December 12, 2024.
Critical Minerals Data Explorer, The International Energy Agency (IEA), May 17, 2024.
Global Critical Minerals Outlook 2024, The International Energy Agency (IEA), May 2024.
No Doubt About It: EVs Really Are Cleaner Than Gas Cars, BloombergNEF, March 21, 2024.
The Largest EV Companies in 2024, The Motley Fool, July 8, 2024.
by Lior Rosenspitz, Jan 2025.